The Enigmatic Neutron Stars: Understanding Stellar Remnants
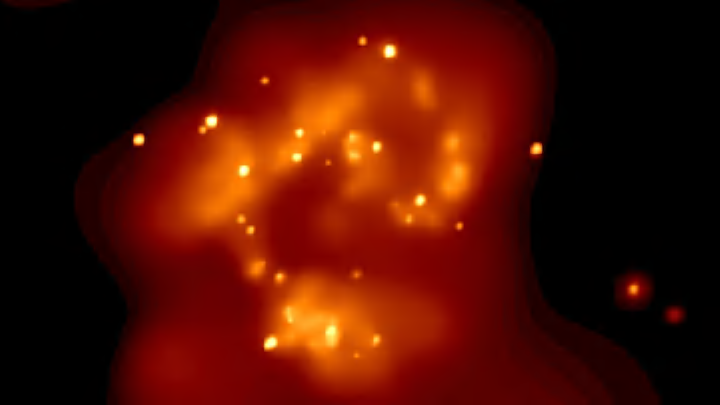
Neutron stars, the incredibly dense remnants of massive stars that have undergone supernova explosions, are among the most fascinating and enigmatic objects in the universe. These compact stellar remnants, with masses comparable to that of the Sun but radii of only about 10 kilometers, challenge our understanding of matter under extreme conditions. Studying neutron stars provides valuable insights into the behavior of matter at nuclear densities, the nature of strong gravitational and magnetic fields, and the processes that govern stellar evolution.
The formation of a neutron star begins with the death of a massive star. When a star with an initial mass greater than about eight times that of the Sun exhausts its nuclear fuel, its core collapses under gravity, and the outer layers are expelled in a supernova explosion. The core, composed primarily of neutrons, is compressed to the point where it becomes a neutron star. This collapse stops when neutron degeneracy pressure, a quantum mechanical effect that arises from the Pauli exclusion principle, counteracts the force of gravity.
Neutron stars are characterized by their extreme density, with masses typically between 1.4 and 2.1 times that of the Sun and densities exceeding that of atomic nuclei. A sugar-cube-sized amount of neutron star material would weigh about a billion tons on Earth. This extreme density leads to unique physical properties and behaviors, making neutron stars natural laboratories for studying matter at nuclear densities and testing the limits of our understanding of fundamental physics.
One of the most remarkable features of neutron stars is their strong magnetic fields, which can be trillions of times stronger than Earth's magnetic field. These magnetic fields influence the behavior of charged particles around the neutron star, leading to the emission of high-energy radiation. In some cases, neutron stars with particularly strong magnetic fields, known as magnetars, can exhibit dramatic and violent outbursts of gamma rays and X-rays, making them some of the most powerful sources of electromagnetic radiation in the universe.
Neutron stars also exhibit rapid rotation, with some rotating hundreds of times per second. These rapidly rotating neutron stars, known as pulsars, emit beams of electromagnetic radiation from their magnetic poles. As the neutron star rotates, these beams sweep across the sky, creating a pulsating effect when observed from Earth. Pulsars were first discovered in 1967 by Jocelyn Bell Burnell and Antony Hewish, and they have since become invaluable tools for studying neutron stars, testing theories of gravity, and probing the interstellar medium.
The study of pulsars has led to numerous important discoveries in astrophysics. For example, the discovery of the first binary pulsar, PSR B1913+16, by Russell Hulse and Joseph Taylor in 1974 provided the first indirect evidence for the existence of gravitational waves. The precise timing of the pulsar's signals revealed the gradual loss of energy from the system, consistent with the emission of gravitational waves predicted by Einstein's general theory of relativity. This discovery earned Hulse and Taylor the Nobel Prize in Physics in 1993.
Another fascinating phenomenon associated with neutron stars is the formation of millisecond pulsars. These are pulsars that have been spun up to very high rotation rates through the accretion of matter from a companion star in a binary system. The transfer of angular momentum from the accreted material causes the neutron star to spin faster, resulting in millisecond pulsars with rotation periods of just a few milliseconds. Studying these systems provides insights into the interactions between neutron stars and their companions, as well as the evolution of binary star systems.
The interiors of neutron stars remain largely mysterious due to the extreme conditions that cannot be replicated in terrestrial laboratories. The equation of state, which describes how matter behaves at such high densities, is a key area of research. Observations of neutron star masses and radii, as well as the detection of gravitational waves from neutron star mergers, provide important constraints on the equation of state and the properties of dense matter.
The detection of gravitational waves from the merger of binary neutron stars by the LIGO and Virgo observatories in 2017 marked a new era in the study of neutron stars. This event, known as GW170817, provided a wealth of information about the behavior of matter at extreme densities, the production of heavy elements through the r-process, and the dynamics of neutron star mergers. The simultaneous observation of electromagnetic counterparts, such as gamma-ray bursts and kilonovae, offered a multi-messenger view of the event, enhancing our understanding of the processes involved.
In conclusion, neutron stars are enigmatic and fascinating objects that provide a unique window into the behavior of matter under extreme conditions. From their formation in supernova explosions to their rapid rotation and strong magnetic fields, neutron stars challenge our understanding of fundamental physics and stellar evolution. Through observations of pulsars, gravitational wave detections, and theoretical modeling, scientists continue to unravel the mysteries of neutron stars, gaining valuable insights into the nature of the universe and the fundamental forces that govern it.